In 2017, Tesla broke new ground by developing a silicon carbide (SiC) drivetrain inverter for their Model 3, becoming the first electric vehicle manufacturer to do so. This was a catalyst for a massive expansion of the SiC industry, which grew from a <$100m market in 2017 to $1bn in 2021, with multiple outlets predicting a CAGR higher than 30% and a $6bn market by 2027. However, on Wednesday 1st March 2023, Tesla made a statement at their investor day that had a major impact on investors in the silicon carbide (SiC) industry.
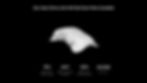
Speaking to the slide in Figure 1 at the Telsa Investor Day, Colin Campbell, VP for Powertrain Engineering, made the following statements about their “next drive unit”:
A new packaging methodology will “extract twice as much heat” compared to an “off the shelf” equivalent, leading to them needing less SiC, which is a major cost reduction.
Their “next drive unit” would have a “75% reduction in silicon carbide”.
The “drive unit cost” has been reduced to “about $1000”.
In typical Tesla style, there was little else. In particular, there was minimal context for the headline grabbing 75% less SiC content. However, the image shown in the slide is what is referred to elsewhere in their presentation as Tesla’s “Next Generation Vehicle”. This has been rumoured to be a mass market vehicle, so could be rated to a much lower power, closer perhaps to the average road vehicle power of circa 100 kW (134 BHP), compared to the higher power 200+ kW (268+ BHP) vehicles that Tesla have produced thus far.
Following the Investor Day, the immediate reaction among investors in SiC companies was a swirl of rumour and concern about the future of a SiC industry that has long been discussed as having a large under-supply for years to come. The notion of a sudden large hole in SiC demand was fuelled by rumours of Tesla reverting to Si IGBTs, hybrid Si and SiC inverters, the adoption of gallium nitride (GaN) technology, and more besides. Immediately, the share price of major SiC companies took a hit. By the morning of the 2nd March, Wolfspeed’s stock had fallen 14% from its previous day’s high, ST Microelectronics’ dropped 5.8%, OnSemi’s 7.3%, and even Aehr Test Systems’ dropped 11%.
Over the next two days, those within the SiC industry got out a calming message, explaining that adjustments to Tesla’s SiC content was expected, and that demand models were not affected by this announcement. By the weekend, the share process of these companies rallied, most returning to a value very similar to that at the start of the week.
In this article, we analyse the first-adopter design of the Model 3 and, using PGC modelling, demonstrate how efficiency savings alone could reduce SiC content by 50%. We will then show how 75% reduction could be achieved in a 110kW car, before addressing some of the more unlikely scenarios and rumours of the following weeks.
Framing Tesla’s 2017 Model 3 SiC Inverter
As the trigger for the SiC boom, the 2017 Model 3 SiC Inverter, seen in Figure 2, is an iconic image in the SiC industry. Numerous articles on this system and widely available teardowns mean that there is plentiful data available on the converter layout and the ST die therein. As a result, it has been pored over by numerous analysts and investors as a means to calculate the SiC content in a vehicle, many of whom have developed a demand model based on a projected number of inverters per processed SiC wafer. However, those inside the industry, including the chip producers themselves, are unlikely to have developed their demand forecasts based on the 2017 Model 3 content, as the technology has taken strides forward in the intervening years.
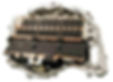
An inverter design will never drive the semiconductor devices to the limits of their thermal ratings, as this would shorten die lifetime and risk failures. By connecting “extra” die in parallel, the current passing through each die is lowered, as is its junction temperature. Therefore, the number of die that are used in parallel, in each of the six switching locations in a drivetrain inverter is a trade-off between the cost of the system on the one hand, versus maximising long term reliability, and increasing efficiency, on the other. A generally accepted rule, adopted herein, is that to maintain safe operation in a SiC inverter, the SiC junction temperature should reach no more than 150°C at the rated inverter power.
As the first automotive adopter of SiC in their 211 kW Model 3, Tesla did not have the luxury of millions of miles of in-product road testing and, as a result, it appears that a reasonably conservative approach was taken to the inverter design to ensure safe operation. Hence, a total of eight 650V STMicroelectronics planar MOSFET die were connected in parallel in each switching location, amounting to a total of 48 die.
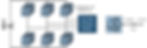
PGC’s proprietary models derive the losses within the die, their resulting temperature of operation, and hence the efficiency of the power converter, all from the inverter layout, the power it is driving, and the type of MOSFETs being employed. Assumptions made within our modelling, regarding thermal resistances, switching speeds and losses, will differentiate our results from the exact Tesla Model 3. However, these have been used to contextualise one scenario in which the use of 8 paralleled ST Gen 2 MOSFET die per switch could be used in a similar automotive inverter. We model two scenarios, the first being at the top-rated 211kW demand. However, this would be an extremely rare occasion, something akin to pulling a caravan up a steep hill or dragging a boat out of water! We therefore also model a constant 50kW demand, which is still a demanding scenario such as hard acceleration, rather than cruising.
In the maximum demand case, our modelling suggests that using eight Gen 2 devices per switching location would limit the temperature rise in each chip (its junction to fluid temperature) to 25°C, while the inverter efficiency would be 99.1%. Assuming a coolant temperature of 75°C, then even under the most extreme stress, the devices will reach just 100°C, far from our safe limit of 150°C. Meanwhile, in the lower 50kW scenario, there would be a temperature rise of around 3°C and an inverter efficiency of 99.6%. While this is very efficient, and extremely reliable, it suggests that the SiC was far from being pushed to its limit.
As well as being a very safe due to its effective derating, the Model 3 design appeared to prioritise accessibility in the event that the newly adopted SiC technology was less reliable than hoped. As seen in Figure 2, rather than opting for a standard design methodology of laying out the die in a closed, optimised module, the SiC components in the Model 3 look like discrete components, the die having been supplied by ST into 24 dual packs. We understand that each dual pack sits on its own direct copper bonded (DCB) substrate, providing an efficient path for heat removal. Despite this, by the rules of conventional inverter design, greater distance between die would typically lead to greater stray inductance and inefficiency. Large bus bars appear to mitigate this, but the whole approach is unique to Tesla and is likely a source of efficiency savings.
OEMs and Tier 1 manufacturers that have subsequently adopted SiC, have made very different design choices, typically adopting power modules in their inverter, very similar to a Si IGBT based inverter. Hyundai/Kia, Lucid and McLaren Applied, for example, have all recently announced that they were using conventional SiC modules supplied by OnSemi’s EliteSiC, Wolfspeed’s XM2 and ST’s ACEPACK, respectively. In another difference, all these systems operate at 800V, instead of Tesla’s 400V.
Finally, the SiC die themselves have moved on a long way since 2017. It was stated by SystemPlus that a device similar to STMicroelectronics’ second generation, automotive qualified SCTW100N65G2AG MOSFET was found in the Tesla Model 3 inverter, though whether it is precisely this device or a bespoke variant is unimportant. Rather, on releasing their 3rd generation of devices, ST announced on powerelectronicnews.com that “with respect to the second generation products […] on-resistance (Ron) x die size FoM has been improved by 30%”. TechInsights put this improvement at 40%. Assessing the Gen 3 range, these new 650V MOSFETs offer 40% lower RON ratings compared to Gen 2 devices at the equivalent current rating. Furthermore, the new devices now have the same current rating at 100°C as at 25°C, where previously the higher temperature rating was 30% less. Meanwhile, switching losses in equivalent current rated devices appear to have reduced by around 50%.
Optimising a Model 3 SiC Inverter
A major step in reducing SiC content in a the Model 3 Inverter will be adopting ST’s latest 3rd Generation MOSFETs, and running them hotter than the Gen 2 devices. As such, we updated our PGC modelling, implementing a 24 die, 211kW automotive inverter, the die parameters updated to reflect the new ST Gen 3 die parameters. All other assumptions in the model were kept the same, including thermal resistance (ignoring for now Tesla’s next gen packaging claim), power factor and switching frequency. The die area of the automotive qualified die was also maintained.
In this “new” four die per switch, 211kW inverter, each die has to take double the current than in the eight die original. As a result, at full load, the losses in each device increase by 93%, as does the junction-to-fluid temperature of each device, which increases to 47°C. Assuming a 75°C coolant line makes the junction temperature 122°C, then even this extreme case remains well below our 150°C safety margin. The resulting inverter efficiency remains similar, 99.1% at the caravan-pulling 211kW full load, and a marginal improvement to 99.7% at 50 kW
Therefore, PGC models suggest that a 50% reduction in SiC MOSFETs, and hence SiC die area, could be achieved by an upgrade from Gen 2 to Gen 3 devices, while accepting marginally higher operating temperatures. Although scant in detail, Tesla’s claim of new packaging methodology extracting “twice as much heat” as any off the shelf device will reduce the (already safe) operating temperatures modelled above, making this scenario more likely.
a 50% reduction in SiC could be achieved by upgrading to Gen 3 devices, while accepting higher operating temperatures
We rule out a scenario in which a 75% reduction in SiC area is achieved without reducing the motor power demand, as 2 MOSFETs per switch would push the current and temperature ratings out of safe usage.
Achieving 75% SiC reduction
While PGC models suggest a 50% SiC die area reduction is possible by upgrading the Tesla Model 3 to a newer design, this falls short of the 75% claim.
At PGC we believe that the simple answer is the most likely. We lean heavily toward the idea that the unrevealed car positioned above the 75% SiC reduction claim in the Tesla Powertrains Slide of Figure 1, is the rumoured mass-market vehicle, with greater territorial reach, and a much small and cheaper motor.
From our model, a motor scaled down to 110kW could be operated using just two die per switch. At exactly 75% reduced SiC in the vehicle, the PGC modelling suggests a 52°C temperature rise within each Gen 3 die, reaching 127°C for an efficiency of 99.1%. Again, the absolute temperature rise could be improved with a next-gen packaging technique.
If each die were in fact 50% larger than they were in Gen 2, an idea suggested in an Aehr Test Systems press release, then a motor of 160kW would satisfy a similar efficiency and temperature rise.
Addressing Other Theories Circulating
Multiple rumours swirled regarding the 75% reduction number. We address a couple of these here.
While we consider all those in this section to be unlikely, the most intriguing would be a shift by Tesla to an 800 V system from its current 400V. This would certainly save SiC die area, a reduction of up to one third for a given power rating. However, Elon Musk and others within Tesla have ruled out a move to 800V in the short term. One reason for this could be that Tesla has an existing network of 480V Superchargers that, in the long-term would need upgrading to 800V. In the short-term, a new 800V Tesla would need to be backwards compatible to the existing network, implying a need for a second on-board charger.
Another proposition is that Tesla could opt for SiC Trench MOSFETs over SiC planar MOSFETs, thereby reducing die area due to the higher power density trench devices offer. We consider this unlikely as the vast majority of SiC devices thus far publicly adopted in drivetrain inverters have been planar MOSFETs, and both of Tesla’s suppliers currently utilise planar designs. For them to produce a first generation of trench devices and immediately supply these to Tesla is unlikely.
A hybrid silicon IGBT / silicon carbide MOSFET approach has significant traction from multiple sources. An idea originating from academic work suggesting that these devices could appear in parallel. While potentially feasible, this approach looks likely to introduce challenging gate driving challenges and could also have reliability concerns.
Rather it is more likely that were Si IGBTs to also be used in this vehicle, they would remain in their current capacity as the devices embedded in a secondary lower power inverter, driving the front wheels in an all-wheel arrangement. One could imagine a small 50kW IGBT based addition to the 110kW /160kW SiC based inverter in the rear. However, as Exawatt rightly point out in their analysis, even low power inverters would payback system-level savings if they adopted SiC.
The final rumour expressed is one of exaggeration and/or a warning shot to SiC OEMs about the on-going cost and undersupply of SiC in the market. This is of course possible and should remind us to view all 2000 words in this piece and the many more elsewhere, with healthy Tesla-based scepticism.
Summary
Mysterious as Tesla’s 75% announcement was, at PGC Consultancy, we are convinced by the simple explanation that this figure is achieved in two stages.
A first 50% reduction can be achieved via efficiency savings achieved by adopting new 3rd Gen ST MOSFETs, implementing efficient packaging and inverter design, and running the devices hotter now we know more of their reliability.
A second 50% reduction in SiC usage is achievable by reducing the motor power from 211kW to 110kW. This reduced power aligns with the idea that this is a cheaper, mass market vehicle that will be more saleable in existing and new territories.